German scientists use supercomputing tech to gain a better understanding of the 3D structure of kilonovae
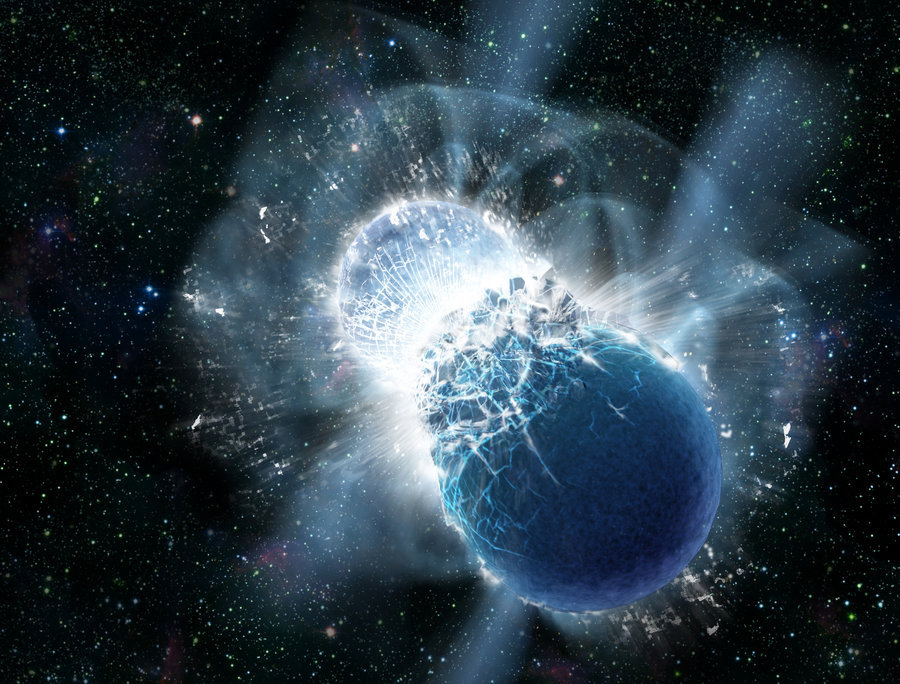
A team of scientists from GSI Helmholtzzentrum für Schwerionenforschung and Queen's University Belfast have produced a 3D supercomputer simulation of the light that is emitted after the merger of two neutron stars, similar to a kilonova that has been observed. The simulation brought together several areas of physics, such as the behavior of matter at high densities, properties of unstable heavy nuclei, and atom-light interactions of heavy elements. This breakthrough in research has provided new insights into the phenomenon of kilonovae.
Recent observations that combine both gravitational waves and visible light have pointed to neutron star mergers as the major site of this element production. According to Luke Shingles, a scientist at GSI/FAIR and the leading author, the unprecedented agreement between their simulations and the observation of kilonova AT2017gfo indicates a broad understanding of the event.
The light that we see through telescopes from the material ejected from a neutron-star merger is determined by the interactions between electrons, ions, and photons within it. Supercomputer simulations of radiative transfer can model these processes and the emitted light. Recently, researchers produced a three-dimensional simulation for the first time that can self-consistently follow the neutron-star merger, neutron-capture nucleosynthesis, energy deposited by radioactive decay, and radiative transfer with tens of millions of atomic transitions of heavy elements.
The 3D model can predict the observed light for any viewing direction. When viewed almost perpendicular to the orbital plane of the two neutron stars, as observational data indicates for the kilonova AT2017gfo, the model predicts a sequence of spectral distributions that look very similar to what has been observed for AT2017gfo. This research area will help us to understand the origins of elements heavier than iron (such as platinum and gold) that were mainly produced by the rapid neutron capture process in neutron star mergers, says Shingles.
Almost half of the elements heavier than iron are produced in an environment of extreme temperatures and neutron densities, as achieved when two neutron stars merge. When they spiral in and coalesce, the resulting explosion leads to the ejection of matter with the appropriate conditions to produce unstable neutron-rich heavy nuclei by a sequence of neutron captures and beta-decays. These nuclei decay to stability, releasing energy that powers an explosive ‘kilonova’ transient, a bright emission of light that rapidly fades in about a week.
The 3D simulation combines several areas of physics, including the behavior of matter at high densities, the properties of unstable heavy nuclei, and atom-light interactions of heavy elements. However, further challenges remain, such as accounting for the rate at which the spectral distribution changes and the description of material ejected at late times. Future progress in this area will increase the precision with which we can predict and understand features in the spectra and will further our understanding of the conditions in which heavy elements were synthesized. High-quality atomic and nuclear experimental data, provided by the FAIR facility, is a fundamental ingredient for these models.